Although lithium ion batteries improved most of these shortcomings, they do have higher risks when it comes to battery failure. Failure in lithium-ion batteries is generally associated with extreme conditions, such as high or low temperatures, overcharge or over-discharge, or a collision or impact that damages the pack.
EV batteries are composed of many hundreds of small, individual battery cells arranged in various configurations. Based on the complexity of the construction and minor differences between cells, it is inevitable that some of the batteries in the pack will experience a minor degree of overcharge or over-discharge. These can usually be modulated by the battery management system (BMS), which is responsible for monitoring and controlling cell balance across the battery and has redundant safety systems in place. However, intentional overcharge or over-discharge cycles through personal use may jeopardize the health and performance of the battery.

Below, we go over some of the possible failure mechanisms in lithium ion batteries. The studies cited below have researched the effects of abusive conditions - low or high temperature, or low or high state of charge - on battery cells without a BMS. In an EV, a lot of these effects are modulated by the presence of thermal and voltage management.
Physical Changes in the Battery
In its simplest form, a battery cell contains three components:
Anode - negative electrode
Cathode - positive electrode
Electrolyte - the medium for ion transfer

In the case of lithium-ion battery discharge, lithium ions flow from the anode to the cathode through an electrolyte solution, forcing electrons to flow around the outside circuit, therefore generating electricity. During charging, the process is reversed, and lithium ions flow from the cathode to the anode.
The process of charging and discharging a battery is not just a chemical or electrical process - it is also a physical one that causes wear and tear on the battery. A lot of this wear and tear is due to microfractures and micro-cracks that form during charging and discharging, and the natural, secondary chemical reactions that happen between battery components. Under ideal conditions, the wear and tear is minimized, and secondary reactions happen slowly. However, many things accelerate the secondary reactions or increase the damaging effects of battery use. For instance, higher voltage charging will increase the force with which lithium goes into the anode, increasing the likelihood of structural damage. High heat will increase the rate of secondary chemical reactions and can accelerate the occurence microfractures.
Charging Risks
Several studies have investigated the physical changes to battery structures during abusive charge and discharge conditions. One major finding was the formation of lithium dendrites in a process called lithium plating (Xu 2012). Lithium dendrites are formed when excess lithium ions accumulate on the anode surface during charging and fail to be absorbed into the anode. This process is not specific to lithium batteries, and also occurs with other metals such as iron and copper.
The formation of dendrites within the battery results in a mismatch of electrical impedance between the anode and cathode, ultimately resulting in short circuits and battery failure (Liu 2014). The well-known Samsung Galaxy Note 7 battery fires of 2016 were a direct result of lithium dendrite growth, which led to internal short circuit and catastrophic battery failure.
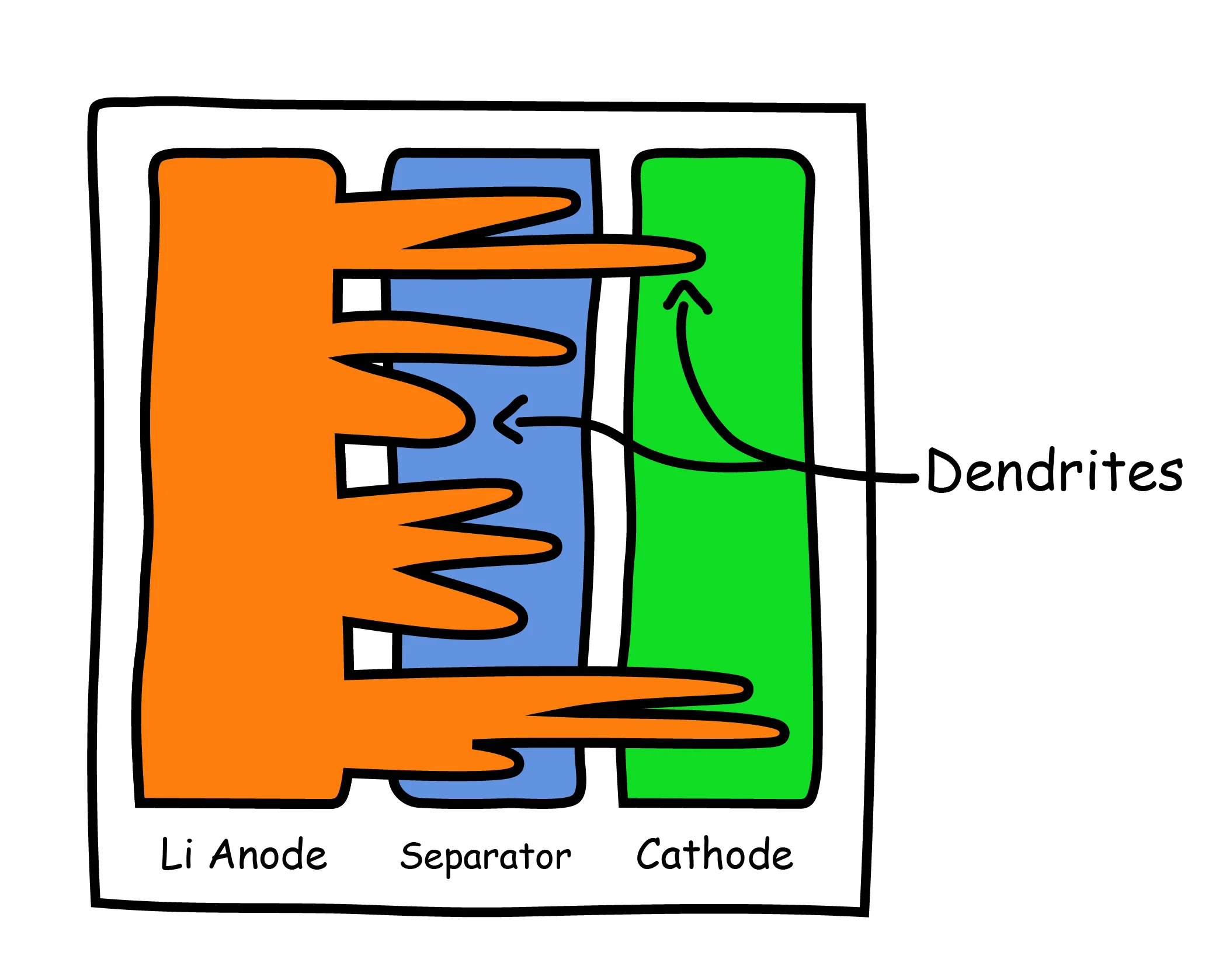
In addition to the possibility of catastrophic failure, dendrites react with the internal electrolyte, causing the solution to decompose, which, over time, will result in a loss of active lithium ions and will deplete the long term capacity.
Discharging Risks
All batteries have something called a solid electrolyte interface (SEI), which is a layer formed by battery materials that are broken down via secondary reactions. While healthy batteries have a base layer of SEI that protects the components, certain conditions such as very low voltage and high heat make it grow even more, causing damage to the battery and using up active materials.
At very low charge levels, the battery may voltage drop to a level that can cause growth of the SEI on the electrodes. In general, voltage drop refers to a loss of electricity to internal resistance. This loss happens all the time, but when the state of charge is low, it can accelerate. When the voltage drop is severe, some of the battery's chemical components can break down and react with the active materials within the cells. These physical changes can lead to a gradual loss of battery capacity. One study indicated that irreversible capacity losses of 12-25% were noted in lithium-based batteries when over-discharging the battery across 1000 cycles (Zhang 2015).
Energy Demands and Aging Characteristics
As electronic devices become more advanced, higher demand is placed on the battery system. Compared to analog devices of the past, which drew a steady current, most digital modern devices load the battery with short, heavy current spikes. For instance, think about how your car battery is used: there are short bursts of energy usage while accelerating or going up hills, but most of the typical usage is lower demand. And, of course, a car is usually parked. With such fluctuating current use, a battery will do best with low internal resistance, which means there is less restriction on the battery to deliver the necessary power when needed. Additionally, high resistance can lead to an inaccurate SoC reading, triggering an inaccurate “low battery” alert as the battery fails to deliver the required energy, despite it being physically available.
The internal resistance of batteries varies with SoC and temperature. Internal resistance of lithium-ion batteries is highest when the battery is empty, and decreases asymptotically until reaching 80-90%, where it begins to increase again.

Therefore, battery performance is most impacted at very low and very high SoC. This issue is further compounded by temperature, which has an even greater effect on internal resistance. Decreasing the temperature from 86F to 14F, for example, can increase internal resistance by up to 30%. Previous studies have shown that abusive charging conditions (low temperatures, extreme discharge or over-charge) led to internal resistances 5 to 7 times greater than that of a fully charged battery (Yuan, 2015).
Finally, as the battery ages, the internal resistance under any conditions increases, decreasing overall battery performance.
Risks
Limited research has been performed to investigate the characteristics of lithium-ion batteries under overcharge or over-discharge failure conditions, specifically with regard to thermal runaway, which is the process that often results in a fire. One study carried out on commercial lithium-ion batteries indicated that after being exposed to abusive conditions, individual cells within the battery respond very differently, likely due to imbalances from operation and manufacturing (Larsson, 2014). This means that the health of the individual cells become more diverse, making it harder for the BMS to determine the overall pack condition. Also, if an individual cell experiences thermal runaway, the entire battery may be at risk.
It is important to note that overcharging in battery studies typically involves taking the SOC beyond 100%, which is hard to do in an EV. Both EV design and the BMS have redundant safety systems in place, including charge and voltage management, and a usable battery capacity that is lower than the total, physical battery capacity.
.webp)
On the other hand, over-discharge is quite achievable - all that needs to happen is that you don’t stop driving your car. Luckily, the fire risk from over-discharge is lower than in the case of overcharging: both the time it took for the battery to ignite and the burn time were significantly longer, but milder in intensity (Ouyang, 2018). Still, don't try to do this!
Aside from the risk of combustion related to battery failure, allowing the EV battery to reach 0% or 100% places significant stress on the battery. Over time, this may result in decreased capacity, which lowers the effective utility of the vehicle, decreases the value, increases the environmental impact, and in extreme cases, could result in battery failure. Occasional complete discharge or complete charge is acceptable, and, as indicated by several manufacturers, may have merit. However, you should minimize the frequency as much as possible, particularly under more extreme conditions.
Written by Brandon August, a lifelong explorer of all things academic. After obtaining an undergraduate physics degree and a doctoral degree in biomedical, he began to explore various professional fields in health and wellness, rideshare work, freelance writing, and day trading. On the recreational side, he has always been involved in the automotive field, owning various vehicles across the years. After a recent move to California, he entered the EV space, purchasing both a Chevrolet Bolt EV and a Bolt EUV for his household.