ICE Vehicle Range
Think back to the last time you purchased a gasoline vehicle. Did you think about the total range of the vehicle, or how far you could travel without stopping? Probably not. Prior to the rise of modern EVs, consumers were primarily concerned with fuel economy (outside of your typical factors such as price, size, and creature comforts). Just look at the advertising for a new Chevrolet Malibu.

In addition to cost and cargo space, fuel economy is a primary detail that we’re provided. To determine the total driving range, we’d have to determine the gas tank size and multiply that by the fuel economy. Interestingly, gas tank size is not commonly reported to consumers, and is a detail that must be found in technical pages for a given vehicle.
In the case of the Malibu shown here, fuel tank capacity is 15.8 gallons. Using the 27 miles per gallon (mpg) provided for city driving, that yields 426.6 miles of drivable range (fuel tank size times mpg). This bumps up to 553 miles on the highway. As of 2021, the median ICE vehicle range was slightly above 400 miles, which is nearly double what the average EV could cover.
EV Range
Now let’s take a look at the advertising for a well-known EV - the Chevrolet Bolt EV. Notice anything different?.

Instead of listing fuel economy, the total range is listed. One thing to note in this advertisement is the lack of battery size details. For vehicles that have several battery size options, such as the Volkswagen ID.4, which comes in the standard 62 kWh battery and an upgraded 82 kWh battery, the battery size is often listed along with the range, allowing fuel efficiency to be calculated. For the Chevrolet Bolt, only one battery size is available, and the battery size must be determined from technical sheets. In fact, not even the original window sticker contains that information.
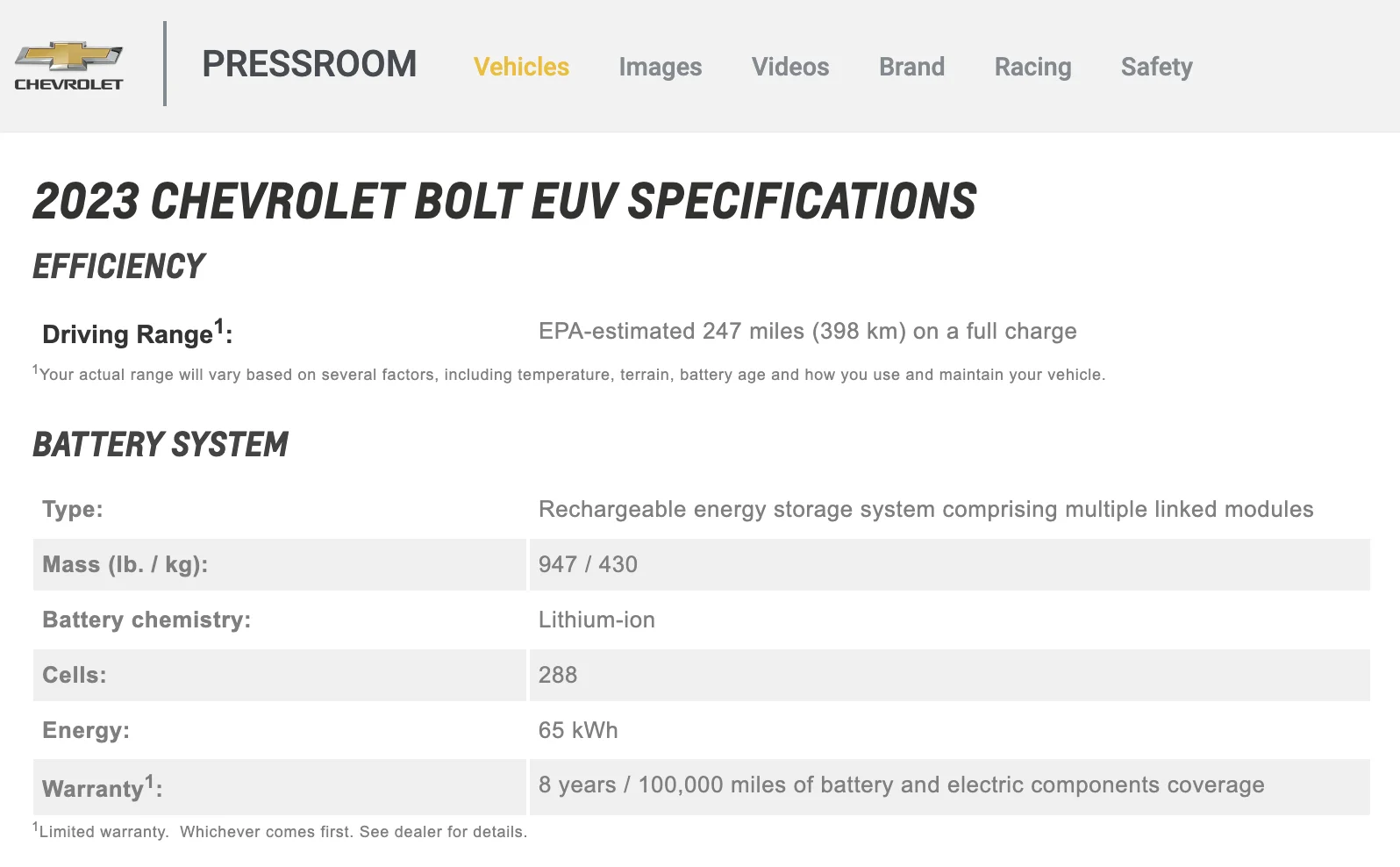
If we look up the specification sheet for the Bolt EV, we see that the new model year vehicles have a 65 kWh battery. Given the total EPA-estimated range of 259 miles, we can determine the expected efficiency: divide the total range by the battery size.
For the Bolt, expected efficiency is just under 4 miles/kWh.
A different measure of efficiency is given when you look at the window sticker for a new Bolt: the EPA MPG-equivalent (or MPGe). This is an efficiency measurement that allows shoppers to compare vehicle fuel efficiency across fuel types. For the Bolt EV, the MPGe is 131 for city driving and 109 for highway driving, with a combined rating of 120. MPGe calculations are derived by using a standard conversion factor between gas and electricity: 1 gallon of gas contains nearly the same energy as 33.7 kWh of electricity. For a deeper dive into MPGe, see our previous article.
It’s easy to see the relationship between MPGe and miles per kWh:
131 MPGe: 131 / 33.7 = 3.89 miles/kWh -> range 252 miles (3.89 mi/kWh x 65 kWh)
109 MPGe: 109 / 33.7 = 3.23 miles/kWh -> range 210 miles
120 MPGe: 120 / 33.7 = 3.56 miles/kWH -> range 231 miles
You can quickly see that these numbers don’t necessarily match what we’re provided from the manufacturer on the vehicle listing. EV manufacturers are continuously pushing for increased EV range, and often list the “best case scenario” range as a way to convince drivers that the range is sufficient for their needs.
What’s the problem with EPA range?
It can get confusing for shoppers if car manufactures display only the EPA-estimated range for an electric car. First, EPA testing is a specific protocol that may or may not match the driving style of a typical consumer. This is particularly true for high speed interstate driving, where EV efficiency, and therefore range, can take a significant hit. Second, manufacturers include an often overlooked disclaimer that states the actual range can significantly vary based on any factors. From Chevrolet’s website: "Actual range may vary based on several factors, including ambient temperature, terrain, battery age and condition, loading, and how you use and maintain your vehicle.” You can read more about the significance of these effects on range.
What Information is Actually Important?
Arguably, range is the most important detail as it will dictate the functionality of the vehicle. For gasoline vehicles, the average gas tank is 12-15 gallons, with the smallest gas tanks being in the 8-10 gallon range. The Scion iQ, for example, has a gas tank of only 8.5 gallons. Given the EPA combined MPG of 37, the vehicle can still travel nearly 315 miles per tank, significantly more than the majority of EVs on the market today.
EVs, on the other hand, vary vastly in battery size and capabilities. Searching for “best EVs” often yields a list of vehicles sorted by highest range, rather than best efficiency. Let’s look at a few examples that vary in battery size and efficiency.
- Mazda MX-30
92 MPGe combined, 35.5 kWh battery
Here’s where EVs get tricky. Although 92 miles per gallon (equivalent) sounds great, this particular EV has a pretty small battery, and thus only has a range of about 100 miles. Regardless how fuel efficient the vehicle is, the range is far too low for most consumers, based on the relatively small battery. In this case, reporting the range is primary, and the efficiency is secondary.
- Hyundai Ioniq 6
140 MPGe combined, 77.4 kWh battery (long range RWD, SE trim)
121 MPGe combined, 77.4 kWh battery (long range RWD, SEL trim)
In this particular case, the efficiency is best in class. For the long range model, the car can be optioned with the SE or SEL trim. For the SE trim, the efficiency and battery size yields a range of nearly 360 miles. The SEL trim, on the other hand, has an estimated range of only 305 miles, despite having an identical battery size. In this case, knowing the efficiency provides some perspective. Interestingly, the major difference between the two trims is the increase in wheel size from 18-inch wheels to 20-inch wheels.
An 11% increase in wheel size results in a 14% decrease in efficiency.
- Hummer EV
53 MPGe combined, 212 kWh battery
Hummers are notorious for two things: huge batteries and being inefficient. The new Hummer EV is no exception. While its range seems impressive at 329 miles, it actually has the largest battery of any EV on the market - a battery that could take much smaller and more efficient EVs much farther. If range is the only thing you’re concerned about, the Hummer EV is a good choice relative to the average EV. However, if you’re factoring in cost to charge, time required for charging, and efficiency, this vehicle makes a very poor choice.
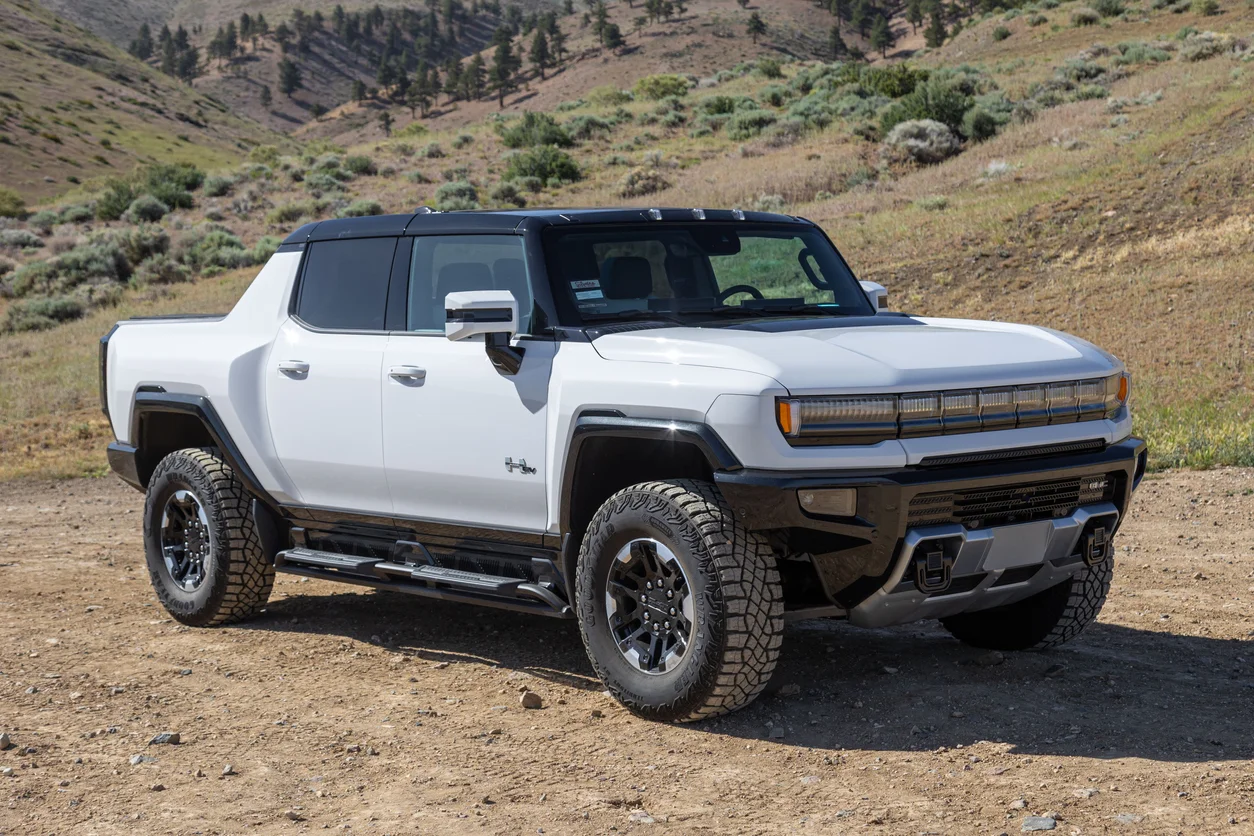
How Should Efficiency Be Reported?
Although MPGe is useful for comparing efficiencies between ICE vehicles and EVs, without a firm understanding of the underlying principles, it does not provide much information for the consumer. A more useful measurement is the amount of energy required to travel for a given distance, or the amount of miles driven per unit of battery energy. Let’s use the Ioniq 6 as an example.
- 24 kWh/100 miles - This is a common measurement reported by the EPA. It is standardized at 100 miles, and describes how many kWh of energy will be consumed to travel this distance.
- 4.17 miles/kWh - This is a common measurement reported via the vehicle’s internal software. It describes how many miles the vehicle can travel for each kWh. Total range can be easily determined by multiplying this number by the battery size. Additionally, this number can be determined by inverting the previous measurement (100 miles / 24 kWh = 4.17 miles/kWh).
Considering that gasoline vehicle efficiencies are listed in miles per gallon, reporting EV efficiency as miles per kWh is, in my opinion, one of the most useful ways. In most cases, it’s unnecessary to directly compare EV to ICE vehicles, as nearly every EV is far more efficient.
MPGe becomes more relevant when determining environmental impact of an EV compared to a gasoline vehicle as a function of use, specifically in relation to material use (e.g. using gasoline and associated emissions). However, given the drastic differences in the way electricity is produced, the measurement often falls short in utility. All gasoline production is “dirty”, whereas increasingly more electricity production is from entirely clean sources and it is technically feasible to power an electric car only with clean energy.
.webp)
TL;DR
Efficiency and range are intricately linked, whether we’re talking about EVs or gasoline vehicles. Higher efficiency does not necessarily mean longer range when we compare different vehicles. Some EVs, like the Nissan Leaf or BMW i3, are considered very efficient, with some reports of 5-6 miles per kWh. However, given the small battery size and very specific, efficient body design, the range is limited, preventing wide-spread adoption. On the other hand, some EVs are less efficient, yielding only 2 to 2.5 miles per kWh (e.g. Rivian R1T), but can still travel 300+ miles to a full charge because of a large, resource-intensive battery.
So why are we so obsessed with EV range? To put it simply - conditioning and routine. The average consumer has been driving gasoline vehicles with an average range of 300+ miles for decades, with little to no concern for gas station availability and reliability. Public EV chargers, on the other hand, can be few and far between, and reliability is mixed.
It’s important to keep in mind that the average driver travels only ~35 miles per day. For older EVs or EVs with very little range, such as the Mazda MX30, having access to home charging, reliable public charging, or charging at work is going to be essential. Additionally, such vehicles make poor road trip vehicles, for obvious reasons. However, this is where efficiency can play a significant role. Keep in mind that efficiency and range are reported as observed by the EPA using a specific protocol. The internet is full of examples of EV owners reporting efficiencies and range far above EPA estimates. In fact, I routinely see 5-6 miles per kWh with my Chevrolet Bolt EUV, which provides a usable range of 300+ miles.